
Journal
of
Physiology
(1990),
423,
pp.
1-14
With
7figure8
Printed
in
Great
Britain
TONIC
VIBRATION
REFLEXES
ELICITED
DURING
FATIGUE
FROM
MAXIMAL
VOLUNTARY
CONTRACTIONS
IN
MAN
BY
L.
G.
BONGIOVANNI*
AND
K.-E.
HAGBARTHt
From
the
Department
of
Clinical
Neurophysiology,
University
Hospital,
S-751
85
Uppsala,
Sweden
(Received
27
April
1989)
SUMMARY
1.
In
the
present
study
on
human
foot
dorsiflexor
muscles
we
have
examined
the
effects
of
high-frequency
(150
Hz)
muscle
vibration
on
weak
or
moderate
voluntary
contractions
(maintained
by
constant
effort)
and
on
maximal
voluntary
contractions
(MVCs)
of
(i)
non-fatigued
muscles,
(ii)
muscles
fatigued
by
sustained
MVCs
and
(iii)
muscles
deprived
of
y-fibre
innervation
by
partial
anaesthetic
nerve
block.
The
motor
outcome
of
the
voluntary
dorsiflexion
efforts
was
assessed
by
measuring
the
firing
rates
of
single
motor
units
in
the
anterior
tibial
(TA)
muscle,
the
mean
voltage
EMG
activity
from
the
pretibial
muscles
and
foot
dorsiflexion
force.
2.
With
the
subject
instructed
to
exert
constant
effort
in
maintaining
a
weak
or
moderate
contraction,
superimposed
vibration
caused
an
enhancement
of
EMG
activity
and
contraction
force.
3.
Previous
claims
that
muscle
vibration
has
no
facilitatory
effect
on
motor
output
in
MVCs
were
found
to
hold
true
for
non-fatigued
but
not
for
fatigued
muscles.
Thus,
the
fatigue-induced
decline
in
EMG
activity
and
motor
unit
firing
rates
was
counteracted
by
short
periods
(<
10-20
s)
of
superimposed
vibration.
However,
with
longer
vibration
periods
it
seemed
as
if
the
initial
facilitation
converted
into
an
opposite
effect
which
accentuated
the
fatigue-induced
decline
in
motor
output
and
contraction
force.
4.
Like
muscle
fatigue,
a
partial
anaesthetic
block
of
the
deep
peroneal
nerve,
supposedly
interrupting
transmission
in
y-motor
fibres,
caused
a
reduction
of
MVC
motor
unit
firing
rates
which
could
be
counteracted
by
muscle
vibration.
In
prolonged
MVCs
performed
during
the
block,
motor
unit
firing
rates
did
not
show
the
normal
progressive
decline
from
an
initially
high
level,
but
stayed
at
a
relatively
constant
low
level
throughout
the
contraction
period.
5.
Even
though
alternative
interpretations
are
possible,
the
results
agree
with
the
hypotheses
(i)
that
in
sustained
MVCs,
fatigue
processes
occur
not
only
in
extrafusal
but
also
in
intrafusal
muscle
fibres,
(ii)
that
the
intrafusal
fatigue
leads
to
a
reduction
of
the
voluntary
drive
conveyed
to
the
ac-motoneurones
via
the
y-loop
and
(iii)
that
vibration-induced
activity
in
group
Ia
afferents
can
act
as
a
substitute
for
the
diminished
fusimotor
drive.
*
Present
address:
Istituto
di
Neurologia,
Universita
degli
Studi
di
Verona,
37126
Verona,
Italy.
t
To
whom
correspondence
and
reprint
requests
should
be
sent.
MS
7658
1
PHY
423
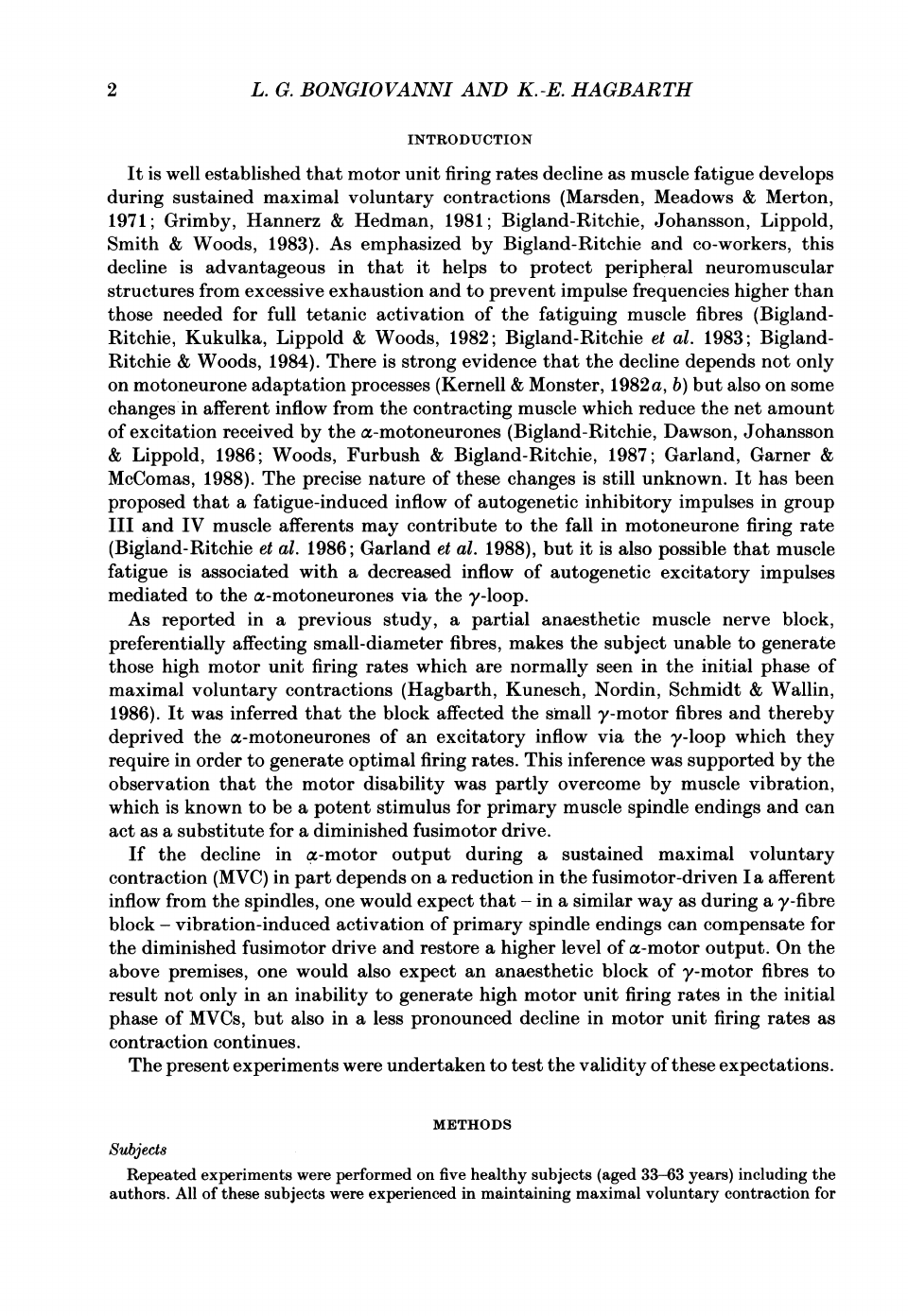
L.
G.
BONGIOVANNI
AND
K.-E.
HAGBARTH
INTRODUCTION
It
is
well
established
that
motor
unit
firing
rates
decline
as
muscle
fatigue
develops
during
sustained
maximal
voluntary
contractions
(Marsden,
Meadows
&
Merton,
1971;
Grimby,
Hannerz
&
Hedman,
1981;
Bigland-Ritchie,
Johansson,
Lippold,
Smith
&
Woods,
1983).
As
emphasized
by
Bigland-Ritchie
and
co-workers,
this
decline
is
advantageous
in
that
it
helps
to
protect
peripheral
neuromuscular
structures
from
excessive
exhaustion
and
to
prevent
impulse
frequencies
higher
than
those
needed
for
full
tetanic
activation
of
the
fatiguing
muscle
fibres
(Bigland-
Ritchie,
Kukulka,
Lippold
&
Woods,
1982;
Bigland-Ritchie
et
al.
1983;
Bigland-
Ritchie
&
Woods,
1984).
There
is
strong
evidence
that
the
decline
depends
not
only
on
motoneurone
adaptation
processes
(Kernell
&
Monster,
1982
a,
b)
but
also
on
some
changes-in
afferent
inflow
from
the
contracting
muscle
which
reduce
the
net
amount
of
excitation
received
by
the
cz-motoneurones
(Bigland-Ritchie,
Dawson,
Johansson
&
Lippold,
1986;
Woods,
Furbush
&
Bigland-Ritchie,
1987;
Garland,
Garner
&
McComas,
1988).
The
precise
nature
of
these
changes
is
still
unknown.
It
has
been
proposed
that
a
fatigue-induced
inflow
of
autogenetic
inhibitory
impulses
in
group
III
and
IV
muscle
afferents
may
contribute
to
the
fall
in
motoneurone
firing
rate
(Bigland-Ritchie
et
al.
1986;
Garland
et
al.
1988),
but
it
is
also
possible
that
muscle
fatigue
is
associated
with
a
decreased
inflow
of
autogenetic
excitatory
impulses
mediated
to
the
a-motoneurones
via
the
y-loop.
As
reported
in
a
previous
study,
a
partial
anaesthetic
muscle
nerve
block,
preferentially
affecting
small-diameter
fibres,
makes
the
subject
unable
to
generate
those
high
motor
unit
firing
rates
which
are
normally
seen
in
the
initial
phase
of
maximal
voluntary
contractions
(Hagbarth,
Kunesch,
Nordin,
Schmidt
&
Wallin,
1986).
It
was
inferred
that
the
block
affected
the
small
y-motor
fibres
and
thereby
deprived
the
a-motoneurones
of
an
excitatory
inflow
via
the
y-loop
which
they
require
in
order
to
generate
optimal
firing
rates.
This
inference
was
supported
by
the
observation
that
the
motor
disability
was
partly
overcome
by
muscle
vibration,
which
is
known
to
be
a
potent
stimulus
for
primary
muscle
spindle
endings
and
can
act as
a
substitute
for
a
diminished
fusimotor
drive.
If
the
decline
in
z-motor
output
during
a
sustained
maximal
voluntary
contraction
(MVC)
in
part
depends
on
a
reduction
in
the
fusimotor-driven
I
a
afferent
inflow
from
the
spindles,
one
would
expect
that
-
in
a
similar
way
as
during
a
y-fibre
block
-
vibration-induced
activation
of
primary
spindle
endings
can
compensate
for
the
diminished
fusimotor
drive
and
restore
a
higher
level
of
a-motor
output.
On
the
above
premises,
one
would
also
expect
an
anaesthetic
block
of
y-motor
fibres
to
result
not
only
in
an
inability
to
generate
high
motor
unit
firing
rates
in
the
initial
phase
of
MVCs,
but
also
in
a
less
pronounced
decline
in
motor
unit
firing
rates
as
contraction
continues.
The
present
experiments
were
undertaken
to
test
the
validity
of
these
expectations.
METHODS
Subject
Repeated
experiments
were
performed
on
five
healthy
subjects
(aged
33-63
years)
including
the
authors.
All
of
these
subjects
were
experienced
in
maintaining
maximal
voluntary
contraction
for
2

TONIC
VIBRATION
REFLEX
IN
MUSCLE
FATIGUE
the
time
required.
Twenty
other
subjects
participated
in
some
of
the
tests.
All
single-motor-unit
recordings
were
made
on
one
of
the
authors
(K.-E.
H.).
The
subjects
gave
their
informed
consent
as
requested
by
the
local
Research
Ethics
Committee.
General
procedures
The
subject
was
comfortably
seated
in
a
semireclining
position
and
the
examined
right
leg
was
fixed
with
the
knee
at
120
deg
and
the
ankle
at
90
deg.
The
foot
was
strapped
to
a
plate
to
which
a
strain
gauge
was
attached
10
cm
from
the
ankle
rotation
axis.
Before
starting
an
experiment,
it
was
checked
that
the
strapping
did
not
cause
any
painful
sensation
from
the
foot
during
maximal
isometric
dorsiflexor
contractions.
Three
types
of
tests
were
designed
to
investigate
the
effect
of
muscle
vibration
on
non-fatigued
and
fatigued
MVCs
of
the
ankle
dorsiflexor
muscles:
(1)
in
a
series
of
brief
(3-4
s)
non-fatigued
MVCs,
control
contractions
alternated
with
contractions
performed
during
concurrent
brief
periods
of
muscle
vibration;
(2)
the
same
type
of
test
was
repeated
after
the
dorsiflexor
muscles
had
been
fatigued
by
a
prolonged
MVC;
(3)
vibration
was
applied
for
20-25
s
while
muscle
fatigue
developed
during
a
sustained
(1
min)
MVC.
Beside
the
vibration-induced
effects
on
MVCs
we
also
studied
the
motor
responses
evoked
when
vibration
was
applied
during
relaxation
and
during
voluntary
dorsiflexor
contractions
of
weak
or
moderate
strength.
The
motor
responses
were
assessed
by
surface
EMG
recordings,
measurements
of
single-motor-unit
firing
rates
and
dorsiflexion
force.
Nerve
block
In
one
experiment,
the
subject
performed
a
series
of
sustained
(1
min)
MVCs
while
he
slowly
recovered
from
a
complete
ankle
dorsiflexor
paresis
induced
by
a
peroneal
nerve
block.
The
block
was
produced
by
injection
of
prilocain
(Citanest
5
mg/ml
+
adrenaline)
around
the
peroneal
nerve
behind
the
fibular
head.
Vibrators
Vibration
was
applied
over
the
tendons
of
the
ankle
dorsiflexor
muscles
using
one
of
two
vibrators
which
were
adjusted
so
that
the
amplitude
of
vibration
was
approximately
1-5
mm
and
the
vibration
frequency
150
Hz.
These
vibrators
were:
(i)
a
commercially
available
electrical
'TVR-vibrator'
(Heiwa
Electronic
Industrial
Co.)
and
(ii)
a
pneumatic
vibrator
powered
by
compressed
air
(to
avoid
electric
artifacts
in
the
electromyograms).
As
in
previous
studies
of
vibration-induced
responses
of
human
muscle
spindle
afferents
(Burke,
Hagbarth,
Lbfstedt
&
Wallin,
1976a,
b)
the
vibrators
were
held
manually
with
a
constant
force
of
application
(20-25
N).
Manual
application
was
superior
to
strapping
the
vibrator
to
the
limb
because
the
strap
could
facilitate
the
spread
of
vibration
to
other
muscles.
Force
measurements
The
strain
gauge
attached
to
the
foot
plate
measured
the
force
resulting
from
contraction
of
the
foot
dorsiflexor
muscles.
Unless
otherwise
stated,
the
subjects
were
not
allowed
to
see
the
signal
from
the
force
transducer
during
the
experiments.
Surface
EMG
This
was
recorded
with
a
pair
of
surface
electrodes
(diameter
0
7
cm)
placed
3
cm
apart
on
the
skin
overlying
the
anterior
tibial
(TA)
muscle
about
15
cm
below
the
knee.
The
signals
were
amplified
(frequency
band
10-100
Hz),
rectified
and
passed
through
a
leaking
integrator
with
a
time
constant
of
0-5
s.
Single-unit
potentials
For
assessment
of
motor
unit
firing
rates
in
the
tibialis
anterior
(TA)
muscle
during
brief
and
sustained
isometric
voluntary
contractions
we
used
electrodes
of
the
type
commonly
used
for
microneurography
(Vallbo,
Hagbarth,
Torebjork
&
Wallin,
1979):
coated
tungsten
electrodes
with
a
shaft
diameter
of
02
mm,
a
tip
diameter
of
5-10
him
and
an
impedance
of
>
100
kQ.
With
a
subcutaneous
reference
needle
electrode
placed
over
the
tibial
bone,
the
microelectrode
was
slowly
advanced
in
the
contracting
muscle
while
searching
for
single-unit
discharges
with
a
high
signal-
to-noise
ratio
and
minor
contamination
from
signals
in
neighbouring
units.
Only
such
recordings
1-2
3

L.
G.
BONGIOVANNI
AND
K.-E.
HAGBARTH
were
accepted
in
which
it
proved
possible
to
follow
the
firing
rates
of
single
identified
units
both
during
brief
and
sustained
MVCs.
Sometimes
two
or
three
units
with
different
spike
amplitudes,
recruitment
thresholds
and
firing
rates
could
be
identified
in
the
same
recording
(Fig.
4).
The
single-unit
data
presented
in
this
and
in
a
succeeding
study
(Bongiovanni,
Hagbarth
&
Stjernberg,
1990)
are
based
on
technically
acceptable
recordings
from
at
least
twenty
motor
units.
Signal
displays
and
analysis
The
signals
were
monitored
on
a
four-channel
storage
oscilloscope
(Tektronix
549)
or
on
a
video
display
monitor
(Gould
V
1000)
and
recorded
on
paper
by
an
electrostatic
recorder
(Gould
ES
1000).
The
plots
showing
single-motor-unit
firing
rates
during
sustained
contractions
were
obtained
by
counting
the
number
of
unitary
spikes/s
with
5
s
intervals
between
each
count.
RESULTS
The
tonic
vibration
reflex
and
its
effect
on
unfatigued
and
fatigued
voluntary
contractions
It
is
well
known
that
sustained
vibration
applied
to
an
initially
relaxed
skeletal
muscle
or
its
tendon
normally
induces
a
tonic
reflex
contraction
in
the
muscle
exposed
to
the
vibration
waves
(Eklund
&
Hagbarth,
1966;
Lance,
De
Gail
&
Neilson,
1966).
Figure
1A
illustrates
a
typical
example
of
such
a
tonic
vibration
reflex
in
the
TA
muscle.
As
shown
by
the
EMG
and
force
traces,
a
weak
contraction
develops
slowly
as
vibration
continues,
and
when
vibration
stops
the
contraction
fades
away
within
a
few
seconds.
EMG
A
Force
20
N
Vibration
EMG
B
--
Force
40
N
a_
I
Vibration
Fig.
1.
TA
surface
EMG
and
force
records
showing
typical
examples
of
the
slow
development
of
the
tonic
vibration
reflex
in
a
resting
subject
(A)
and
the
abrupt
onset
of
the
reflex
in
a
subject
asked
to
exert
constant
effort
in
the
maintenance
of
a
weak
voluntary
background
contraction
(B).
In
this,
as
in
the
succeeding
figures,
filled
bars
indicate
vibration
at
150
Hz
applied
over
the
TA
tendon.
It
is
also
known
from
previous
reports
that
when
vibration
is
applied
to
a
muscle
engaged
in
a
weak
or
moderate
voluntary
contraction
(maintained
by
constant
effort),
the
vibration
reflex
produces
an
involuntary
enhancement
of
a-motor
output
(EMG)
and
contraction
strength
(Hagbarth
&
Eklund,
1966;
McCloskey,
Ebeling
&
Goodwin,
1974).
We
observed
such
vibration-induced
enhancements
of
weak
or
moderate
voluntary
contractions
in
all
five
subjects
exposed
to
this
test,
and
an
example
is
shown
in
Fig.
1B
(same
subject
as
in
Fig.
1A).
In
comparison
with
the
4

TONIC
VIBRATION
REFLEX
IN
MUSCLE
FATIGUE
reflex
elicited
in
an
initially
relaxed
muscle,
the
response
to
vibration
was
stronger
and
had
a
more
abrupt
onset
when
displayed
against
the
background
of
a
weak
voluntary
contraction
(note
different
force
scales
in
Fig.
1A
and
B).
Once
a
tonic
reflex
response
had
reached
its
plateau
level,
this
level
stayed
constant
during
the
rest
of
the
vibration
period
(longest
vibration
period
30
s).
A
B
EMG
4
min
of
MWC
ForceJJJJJJ
LL
~~
)
~
~
1111
ll
I
200
N
SLA~~~iN
I
I
I
I
I
I
I
20
s
Fig.
2.
EMG
activity
and
force
developed
by
foot
dorsiflexor
muscles
during
brief
MVCs
repeated
with
rest
intervals
of
about
20
s
and
with
vibration
applied
to
the
TA
tendon
during
every
second
contraction.
A,
in
the
non-fatigued
subject,
the
EMG
and
force
traces
are
similar
in
contractions
with
and
without
vibration.
B,
with
the
subject
fatigued
by
a
preceding
prolonged
MVC,
vibration
has
a
facilitatory
effect
on
the
brief
MVCs
and
counteracts
the
fatigue-induced
decline
in
EMG
activity
and
force.
See
text.
According
to
previous
reports,
muscle
vibration
does
not
normally
enhance
motor
output
in
maximal
voluntary
contractions
(Hagbarth
&
Eklund,
1969;
Hagbarth
et
al.
1986).
In
the
present
study,
this
was
found
to
hold
true
for
unfatigued
but
not
for
fatigued
MVCs.
The
procedure
illustrated
in
Fig.
2A
was
adopted
to
test
the
effect
of
muscle
vibration
on
EMG
activity
and
force
in
unfatigued
MVCs.
After
a
period
of
relaxation,
lasting
for
at
least
3
min,
the
subject
initiated
a
series
of
brief
(3-4
s)
MVCs,
separated
by
rest
periods
of
20
s.
Control
contractions
(without
vibration)
were
alternated
with
contractions
performed
while
vibration
was
applied
to
the
TA
tendon.
No
sign
of
a
tonic
vibration
reflex
could
be
detected
in
any
of
the
twenty-
five
subjects
exposed
to
this
test,
i.e.
vibration
had
no
significant
influence
on
ax-motor
output
(EMG)
and
contraction
force
(example
shown
in
Fig.
2A).
In
the
experiment
illustrated
in
Fig.
2,
the
series
of
intermittent
MVCs
was
temporarily
interrupted
by
a
long-lasting
(4
min),
fatiguing
MVC.
Following
this
manoeuvre,
there
was
a
marked
reduction
of
the
EMG
activity
recorded
during
the
intermittent
MVCs
(mean:
-18
%),
and
this
fatigue-induced
decline
in
motor
output
was
to
a
large
extent
compensated
for
by
vibration.
In
the
sequence
shown
in
Fig.
2B,
the
EMG
peaks
were
on
average
16
%
higher
in
the
vibration-exposed
than
in
the
interspersed
control
contractions,
whereas
the
vibration-induced
enhancement
of
contraction
force
did
not
amount
to
more
than
4
%.
Four
experiments
of
this
type
were
performed
(on
two
subjects)
and
they
all
showed
that
vibration
effectively
counteracted
the
fatigue-induced
decline
in
EMG.
activity
with
little
or
no
corresponding
effect
on
the
force.
5

L.
G.
BONGIOVANNI
AND
K.-E.
HAGBARTH
More
complex
responses
were
seen
when
vibration
was
applied
during
sustained
MVCs.
Figure
3A
shows
the
well-known
progressive
decline
in
EMG
activity
and
force,
normally
occurring
during
a
sustained
MVC.
In
five
subjects,
the
TA
tendon
was
exposed
to
vibration
for
about
25
s
during
the
course
of
such
a
sustained
MVC,
EMG
A
Force
200
N
20
s
EMG
Force
200
N
20
s
Vibration
Fig.
3.
A,
a
sustained
(60
s)
MVC
of
the
foot
dorsiflexor
muscles
showing
the
typical
progressive
decline
of
EMG
activity
and
force.
B,
effects
of
20
s
vibration
during
a
similar
contraction
performed
by
the
same
subject.
Note
the
initial
facilitatory
effect
(most
prominent
in
the
EMG
trace)
with
succeeding
rapid
decline
and
post-vibratory
recovery.
See
text.
and
Fig.
3B
shows
a
representative
example
of
the
resulting
changes
in
EMG
activity
and
force.
The
EMG
activity
rose
during
the
initial
phase
of
vibration,
but
as
vibration
continued
this
initial
increase
was
followed
by
a
rapid
decline.
Cessation
of
vibration
was
followed
by
post-vibratory
enhancement
of
EMG
activity.
In
the
example
illustrated,
the
initial
increase
in
EMG
activity
had
no
distinct
counterpart
in
the
force
trace,
whereas
the
down-going
component
of
the
response
and
the
post-
vibratory
recovery
was
also
observed
in
this
trace.
A
vibration-induced
motor
response
of
similar
shape
as
seen
in
the
surface
EMG
records
was
observed
also
when
studying
the
firing
rates
of
individual
TA
motor
units
during
sustained
MVCs.
However,
it
was
noticed
that
different
motor
units
were
not
affected
to
the
same
degree
by
vibration.
The
units
which
were
most
susceptible
to
the
influence
of
muscle
vibration
in
these
tests
were
those
which
were
characterized
by
(1)
a
high
recruitment
threshold
in
voluntary
isometric
contrac-
tions,
(2)
a
high
firing
rate
during
the
initial
phase
of
MVCs
and
(3)
a
rapid
decline
in
firing
rate
during
sustained
MVCs.
These
high-threshold
units
were
not
activated
by
vibration
alone,
i.e.
they
were
not
recruited
when
the
tonic
vibration
reflex
was
elicited
in
an
initially
relaxed
muscle.
By
contrast,
low-threshold
units,
which
were
recruited
both
by
the
vibration
reflex
alone
and
by
weak
voluntary
contractions,
were
relatively
more
resistant
to
the
influence
of
vibration
while
fatigue
developed
during
sustained
MVCs.
Figure
4
illustrates
our
way
of
classifying
'low-threshold'
and
'high-threshold'
units,
and
Fig.
5
presents
an
example
of
how
the
two
extreme
6

TONIC
VIBRATION
REFLEX
IN
MUSCLE
FATIGUE
types
of
units
responded
to
vibration
as
muscle
force
declined
during
a
sustained
MVC.
Both
units
responded
with
a
transient
initial
increase
in
firing
rate,
more
pronounced
for
the
high-threshold
than
for
the
low-threshold
unit,
and
in
this
case
also
the
force
record
showed
an
initial
up-going
deflection.
However,
as
vibration
continued,
the
initial
facilitatory
effect
vanished
and
seemed
to
be
followed
by
an
effect
which
acted
in
the
opposite
direction
and
which
was
followed
by
a
post-
vibratory
rebound.
I
I
II
I
li
ii
ii
iii
II
II
/>
~~-~~~-~~=
-
I20
N
A~~~~~~~~~~~~~~
is
F
T
15
B~~~~~~~~~~~~~~~~~~~~~~~
1
50
N
is
Fig.
4.
Differentiation
between
'low-threshold'
and
'high-threshold'
motor
units
in
the
TA
muscle.
A,
example
of
a
'low-threshold'
unit
being
recruited
by
the
tonic
vibration
reflex
elicited
in
a
resting
subject.
Firing
starts
at
a
minimal
dorsiflexion
force
(
<
3
N).
B,
the
same
unit
is
recruited
at
a
similar
low
dorsiflexion
force
during
a
slowly
rising
voluntary
isometric
contraction.
A
second
unit
comes
into
play
at
about
40
N
and
a
third
unit
at
about
100
N.
Units
of
the
latter
type,
firing
only
when
force
exceeds
50%
of
(non-
fatigued)
maximal
contraction
strength,
are
in
this
and
the
succeeding
study
(Bongiovanni,
Hagbarth
&
Stjernberg,
1990)
classified
as
'high-threshold'
units.
(Low
background
noise
eliminated
by
window
discriminator.)
Figure
6
shows
another
example
of
a
high-threshold
motor
unit
with
a
rapid
fall
in
firing
rate
during
a
sustained
MVC.
In
this
case,
vibration
was
applied
at
a
stage
when
the
unit
had
ceased
firing
to
the
voluntary
drive.
The
vibration
reflex
brought
the
unit
back
into
activity,
and
this
improvement
in
motor
performance
was
reflected
also
in
the
force
trace.
It
should
be
noticed
that
whenever
vibration
was
applied
during
the
course
of
a
sustained
MVC
the
initial
facilitatory
effect
of
vibration
was
never
potent
enough
to
fully
compensate
for
the
fatigue-induced
decline
in
motor
unit
firing
rates.
It
should
also
be
pointed
out
that the
high
motor
unit
firing
rates
seen
in
the
unfatigued
muscle
during
brief
MVCs
or
at
the
very
beginning
of
a
sustained
MVC
could
not
be
enhanced
further
by
vibration.
Nerve
block
experiment
After
injection
of
the
anaesthetic
drug
there
was
a
total
paresis
of
the
foot
dorsiflexor
muscles
lasting
for
about
3
h.
Then,
it
took
about
65
min
from
the
moment
when
the
first
EMG
signs
of
motor
unit
activity
reappeared
in
the
TA
muscle
until
the
subject
regained
full
contraction
strength.
While
the
subject
slowly
7

L.
G.
BONGIOVANNI
AND
K.-E.
HAGBARTH
N
I
4)
40
CD
._
0.
.
&P
L-
0
0
30-
20
10
*
High-threshold
motor
unit
o
Low-threshold
motor
unit
0
i
I
.
.
.
.
.
.
.
I
*
I
.
.
.
.
.
100
S
Vibration
Fig.
5.
Example
showing
that
the
vibration
reflex
temporarily
counteracts
the
progressive
decline
in
firing
rates
of
a
high-
and
a
low-threshold
TA
motor
unit
during
a
sustained
MVC.
Note
that
the
initial
facilitatory
effect
of
vibration
-
more
pronounced
for
the
high-
threshold
unit
-
is
succeeded
by
a
rapid
fall
in
firing
rate
and
a
post-vibratory
rebound.
Similar
biphasic
responses
to
vibration
are
seen
also
in
the
force
trace.
N
I
4)
40
._
0)
.
tr_
L-
0
LL
40
-
30*
20
3001
250
200
150
Vibration
-I
10os
Fig.
6.
High-threshold
TA
motor
units
with
a
high
initial
firing
rate
which
rapidly
declines
during
a
sustained
MVC.
The
unit
stops
discharging
after
55
s,
but
is
brought
back
into
action
when
vibration
is
applied
to
the
TA
tendon.
Note
also
concomitant
rise
in
force.
8

TONIC
VIBRATION
REFLEX
IN
MUSCLE
FATIGUE
recovered
from
the
nerve
block
we
examined
several
times
his
ability
to
maintain
maximal
voluntary
dorsiflexor
contractions
for
1
min.
Figure
7A
shows
the
way
in
which
the
firing
rate
of
a
single
motor
unit
in
the
TA
muscle
changed
from
one
contraction
to
the
next
as
the
recovery
proceeded.
During
the
first
contraction,
0
20
min
a
25
min
*29
min
A
A
65
min
-W
40-
co
E
30
00:
It3
j B|
8
0
30
60s
30
-0
4-.
o
CD
I
20
0
4
z
eoo
o
ii
aN
Hliuai
|I
,
20
s
Vibration
Fig.
7.
MVCs
performed
during
65
min
of
recovery
from
a
complete
peroneal
nerve
block.
A,
firing
patterns
of
a
single
TA
motor
unit
during
four
sustained
(60
s)
MVCs
performed
at
different
stages
of
recovery.
Note
that
in
the
early
recovery
phase
(20
min)
the
firing
rate
stays
at
about
the
same
low
level
throughout
the
contraction
period,
but
as
recovery
proceeds
and
the
initial
firing
rate
rises,
the
frequency
drop,
normally
associated
with
fatigue,
becomes
progressively
more
pronounced.
B,
recordings
from
another
TA
motor
unit
obtained
after
7
min
of
recovery.
Upper
diagram
depicts
the
peak
firing
rate
of
this
unit
during
recurrent
brief
(3-4
s)
MVCs
separated
by
rest
intervals
of
20
s.
Besides
showing
a
gradual
increase
in
firing
rate
and
force
as
recovery proceeds
(during
5
min),
the
diagram
illustrates
the
facilitatory
effect
of
the
vibration
reflex.
initiated
after
20
min
of
recovery,
the
firing
rate
varied
within
the
range
of
20-24
Hz
and
did
not
show
any
obvious
decline.
As
time
passed,
there
was
a
progressive
increase
in
the
firing
rates
during
the
initial
phases
of
the
MVCs,
and
at
the
same
time
the
decline
in
firing
rate
during
the
sustained
contractions
became
more
pronounced.
Hence,
the
discharge
rates
at
the
end
of
the
contraction
periods
were
relatively
similar
and
independent
of
the
initial
firing
levels.
Also
the
accompanying
force
trace
showed
that
the
downward
slopes
became
steeper
as
the
initial
peak
values
rose
during
the
period
of
recovery
(not
illustrated).
The
effect
of
vibration
on
motor
performance
was
examined
during
the
first
20
min
of
recovery
(i.e.
before
the
sequence
illustrated
in
Fig.
7A).
During
this
early
period
of
recovery
the
subject
was
instructed
to
make
brief
(3-4
s)
intermittent
MVCs,
separated
by
rest
periods
of
20
s.
As
long
as
maximal
dorsiflexion
force
was
less
than
10
%
of
normal
values,
vibration
applied
over
the
TA
tendon
had
no
apparent
effect
I
9

L.
G.
BONGIOVANNIVI
AND
K.-E.
HAGBARTH
on
motor
performance,
but
a
facilitatory
effect
appeared
at
the
stage
of
recovery
when
the
subject
had
regained
about
20%
of
his
normal
dorsiflexion
force.
Such
a
vibration-induced
improvement
of
motor
performance
is
illustrated
in
Fig.
7B
where
the
peak
dorsiflexion
force
and
the
firing
rate
of
a
single
TA
motor
unit
are
depicted
for
each
of
eighteen
successive
contractions.
DISCUSSION
Even
though
alternative
interpretations
cannot
be
definitely
excluded,
the
results
are
for
the
most
part
in
accordance
with
the
hypothesis
that
the
decline
in
motor
unit
firing
rates
during
sustained
MVCs
in
part
depends
on
a
reduction
in
fusimotor-
driven
I
a
afferent
inflow
from
the
spindles.
From
previous
microneurographic
recordings
in
man
it
is
known
that
muscle
vibration
of
the
type
used
in
the
present
study
(150
Hz,
amplitude
1f5
mm)
gives
rise
to
an
intense,
sustained
discharge
in
group
Ia
afferents
(Burke
et
al.
1976a,
b),
which
resembles
the
discharge
which
can
be
evoked
by
fusimotor
activation
of
primary
spindle
endings
during
isometric
voluntary
contractions
(Vallbo,
1973;
Vallbo
et
al.
1979).
With
increasing
strength
of
a
voluntary
isometric
contraction,
the
afferent
feedback
from
the
muscle
spindles
in
the
contracting
muscle
grows
as
an
increasing
number
of
I
a
afferents
are
recruited
into
action
(Burke,
Hagbarth
&
Skuse,
1978)
and
the
firing
rate
of
individual
I
a
units
is
enhanced
(Vallbo,
1974).
The
upper
limit
for
the
I
a
firing
rate
in
MVCs
has
for
technical
reasons
been
difficult
to
assess
(neurogram
contaminated
by
EMG
signals),
but
firing
rates
close
to
100
Hz
have
been
observed
in
voluntary
contractions
of
moderate
strength
(Vallbo,
1973)
and
this
approaches
the
highest
steady
firing
rates
observed
in
response
to
vibration
(Burke
et
al.
1976
a
b).
In
the
present
study,
we
used
an
indirect
way
of
assessing
changes
in
afferent
inflow
from
the
primary
spindle
endings
as
muscle
fatigue
developed.
The
technique
is
based
on
the
presumption
that
by
studying
the
facilitatory
effects
of
muscle
vibration
on
a-motor
output
in
voluntary
contractions
of
different
strength
it
is
possible
to
make
an
appraisal
of
the
background
fusimotor-driven
I
a
discharge
in
these
contractions.
The
fact
that
vibration
enhances
the
motor
output
in
weak
or
moderate
contractions
(Fig.
1
B)
may
be
taken
as
an
indication
that
vibration
induces
a
I
a
afferent
inflow
which
exceeds
the
pre-existing
fusimotor-driven
discharge
and
thereby
allows
the
tonic
vibration
reflex
to
exert
its
autogenetic
excitatory
effect
on
the
a-motoneurones.
As
the
fusimotor-driven
discharge
increases
with
increasing
contraction
strength,
a
stage
will
probably
be
reached
when
vibration
can
cause
little
or
no
further
enhancement
of the
I
a
inflow.
Such
a
state
of
'saturation'
may
well
account
for
the
fact
that
vibration
has
no
facilitatory
effect
on
EMG
activity
and
motor
unit
firing
rates
in
unfatigued
MVCs.
However,
as
shown
in
the
present
study
vibration
regains
its
autogenetic
excitatory
effect
on
the
motor
output
when
muscle
fatigue
develops
during
intermittent
or
maintained
MVCs
(Figs
2B,
3B,
5
and
6).
The
simplest
explanation
of
this
finding
is
that
muscle
fatigue
from
MVCs
is
associated
with
such
a
decline
in
the
fusimotor-driven
I
a
discharge
that
the
vibration
response
is
no
longer
occluded.
The
present
results
provided
no
definite
clue
regarding
the
mechanisms
responsible
for
the
deduced
decline
in
Ia
afferent
activity.
It
is
possible
that
in
sustained
MVCs
to

TONIC
VIBRATION
REFLEX
IN
MUSCLE
FATIGUE
fatigue
processes
occur
not
only
in
extrafusal
but
also
in
intrafusal
muscle
fibres,
and
that
a
fatigue-induced
decline
in
intrafusal
contraction
force
leads
to
a
reduction
in
the
fusimotor-driven
afferent
discharge.
Signs
of
such
intrafusal
fatigue
have
been
observed
following
prolonged
stimulation
of
static
y-axons
in
the
cat,
and
there
is
evidence
that
reduction
of
muscle
blood
flow
(which
occurs
during
sustained
MVCs)
enhances
muscle
spindle
fatigue
(Barker,
Emonet-Denand,
Harker,
Jami
&
Laporte,
1976;
Emonet-Denand
&
Laporte,
1978;
Decorte,
Emonet-Denand,
Harker,
Jami
&
Laporte,
1984).
An
alternative
possibility
is
that
muscle
fatigue
gives
rise
to
an
inhibitory
inflow
in
group
III
and
IV
muscle
afferents
(Bigland-Ritchie
et
al.
1986;
Garland
et
al.
1988)
which
lowers
the
discharge
rates
not
only
in
a-
but
also
in
y-motoneurones
and
thereby
reduces
the
fusimotor
activation
of
the
spindle
endings
(cf.
comments
below
regarding
the
outcome
of
the
present
nerve
block
experiment).
During
sustained
MVCs,
the
amount
of
excitation
that
the
motoneurones
receive
from
their
own
muscle
may
be
reduced
not
only
by
intrafusal
fatigue
but
also
by
such
mechanisms
as
presynaptic
inhibition
of
Ia
terminals
or
'transmitter
depletion'
at
Ia
synapses
(Hagbarth
&
Eklund,
1966;
De
Gail,
Lance
&
Neilson,
1966;
Delwaide,
1973;
Desmedt
&
Godaux,
1978;
Hultborn,
Meunier,
Morin
&
Pierrot-Deseilligny,
1987
a).
According
to
Hultborn,
Meunier,
Pierrot-Deseilligny
&
Shindo
(1987b)
there
is
at
the
onset
of
a
voluntary
contraction
a
decrease
in
presynaptic
inhibition
of
I
a
terminals
on
the
motoneurones
of
the
contracting
muscle.
If
this
'opening'
of
I
a
transmission
subsides
as
contraction
continues,
the
motoneurone
firing
rate
will
decline.
Motoneurone
adaptation
is
probably
another
factor
contributing
to
the
progressive
fall
in
motoneurone
firing
rates
(Kernell
&
Monster,
1982
a,
b)
and
Renshaw
inhibition
may
also
be
involved
in
this
process.
In
agreement
with
previous
observations
by
Grimby
et
al.
(1981)
we
noted
that
high-threshold
motor
units
with
high
initial
firing
rates
during
MVCs
show
a
faster
frequency
drop
during
fatigue
than
low-threshold
units.
This
seems
to
accord
with
findings
in
the
cat
that
late
adaptation
is
more
prominent
in
large
motoneurones
than
in
small
ones,
and
that
for
one
and
the
same
cell
adaptation
is
more
pronounced
at
high
than
at
lower
initial
firing
rates
(Kernell
&
Monster,
1982a,
b).
However,
it
is
also
to
be
expected
that
the
last
recruited
motor
units
are
the
first
to
show
a
frequency
drop
and
to
be
de-recruited
as
the
excitatory
drive
on
the
motoneurone
pool
declines.
In
similar
terms,
it
is
also
possible
to
explain
why
the
facilitatory
effect
of
vibration
was
more
pronounced
on
the
high-threshold
than
on
the
low-threshold
units
(Fig.
5).
Merton
(1954)
and
Bigland-Ritchie,
Jones
&
Woods
(1979)
have
reported
that
during
sustained
MVCs,
performed
by
well-trained
and
motivated
subjects,
superimposed
electrical
tetanization
of
the
muscle
nerve
increases
EMG
activity
but
not
tension,
indicating
that
the
reduction
in
motor
unit
firing
rate
is
not
responsible
for
loss
of
force.
However,
less
well
trained
or
motivated
subjects
may
have
considerable
difficulty
in
maintaining
a
voluntary
drive
sufficient
to
prevent
supramaximal
nerve
stimuli
from
causing
an
enhancement
of
contraction
strength
(Grimby
et
al.
1981;
Woods
et
al.
1987).
Different
degrees
of
motivation
in
the
maximal
force
tasks
may
well
account
for
the
fact
that
the
vibration-induced
enhancement
of
motor
output
during
sustained
MVCs
was
sometimes
but
not
always
accompanied
by
a
distinct
increase
in
contraction
force
(cf.
Figs
3B
and
6).
If
a
reflex
inhibition
of
a-motoneurones
from
group
III
and
IV
muscle
afferents
is
11

L.
G.
BONGIOVANNI
AND
K.-E.
HAGBARTH
a
prime
cause
of
the
decline
in
motor
unit
firing
rates
during
sustained
MVCs
(Bigland-Ritchie
et
al.
1986;
Garland
et
al.
1988),
one
would
expect
an
anaesthetic
block
of
these
small-diameter
fibres
to
enhance
the
subject's
ability
to
maintain
high
motor
unit
firing
rates.
This
did
not
happen
in
the
nerve
block
experiment.
The
outcome
of
this
experiment
indicates
that
a
block
of
small-diameter
fibres
in
the
muscle
nerve
removes
an
excitatory
inflow
to
the
a-motoneurones
which
is
needed
for
the
generation
of
the
high
initial
firing
rates
in
MVCs,
and
which
fades
away
as
muscle
fatigue
develops.
Without
ascribing
all
the
effects
of
the
local
anaesthetic
as
seen
in
the
EMG
to
a
y-fibre
block,
our
tentative
interpretation
of the
results
presented
in
Fig.
7A
is
that
an
anaesthetic
block
affecting
the
efferent
link
of
the
y-loop
results
in
a
similar
disability
to
generate
high
motor
unit
firing
rates
as
is
normally
encountered
when
y-loop
support
has
faded
away,
partly
because
of
muscle
spindle
fatigue,
at
the
end
of
a
long-lasting
MVC.
However,
the
results
do
not
exclude
the
possibility
that
a
reflex
inhibition
of
y-motoneurones
by
small
diameter
muscle
afferents
contributes
to
the
fatigue-induced
decline
in
y-loop
support.
At
any
rate,
the
observations
indicate
that
the
y-loop
serves
an
important
function
in
adjusting
motor
unit
firing
rates
to
the
changes
in
the
contractile
characteristics
of
fatiguing
extrafusal
muscle
fibres.
It
is
of
interest
to
note,
that
a
similar
disability
to
generate
high
initial
motor
unit
firing
rates,
as
illustrated
by
the
bottom
traces
in
Fig.
7A,
was
observed
by
Burke,
Gandevia,
Macefield
&
McKenzie
(1988)
who
recorded
from
motor
axons
proximal
to
a
complete
nerve
block
during
prolonged
MCVs.
An
unexpected
finding
in
the
present
study
was
that,
with
vibration
periods
lasting
more
than
10-20
s
during
MVCs,
the
initial
facilitatory
effect
of
vibration
often
turned
into
an
opposite
effect,
which
seemed
to
accentuate
the
fatigue-induced
decline
in
EMG
activity
and
motor
unit
firing
rates
(Figs
3B
and
5).
This
late
effect
of
prolonged
muscle
vibration
will
be
further
dealt
with
in
a
separate
report
(Bongiovanni
et
al.
1990).
This
work
was
supported
by
the
Swedish
Medical
Research
Council,
grant
No.
B89-14X-02881-
20C.
REFERENCES
BARKER,
D.,
EMONET-DANAND,
F.,
HARKER,
D.
W.,
JAMI,
L.
&
LAPORTE,
Y.
(1976).
Distribution
of
fusimotor
axons
to
intrafusal
muscle
fibres
in
cat
tenuissimus
spindles
as
determined
by
the
glycogen-depletion
method.
Journal
of
Physiology
261,
49-69.
BIGLAND-RITCHIE,
B.
R.,
DAWSON,
N.
J.,
JOHANSSON,
R.
S.
&
LTPPOLD,
0.
C.
J.
(1986).
Reflex
origin
for
the
slowing
of
motoneurone
firing
rates
in
fatigue
of
human
voluntary
contractions.
Journal
of
Physiology
379,
451-459.
BIGLAND-RITCHIE,
B.,
JOHANSSON,
R.,
LIPPOLD,
0.
C.
J.,
SMITH,
S.
&
WOODS,
J.
J.
(1983).
Changes
in
motoneurone
firing
rates
during
sustained
maximal
voluntary
contractions.
Journal
of
Physiology
340,
335-346.
BIGLAND-RITCHIE,
B.,
JONES,
D.
A.
&
WOODS,
J.
J.
(1979).
Excitation
frequency
and
muscle
fatigue:
electrical
responses
during
human
voluntary
and
stimulated
contractions.
Experimental
Neurology
64,
414-427.
BIGLAND-RITCHIE,
B.,
KUKULKA,
C.
G.,
LIPPOLD,
0.
C.
J.
&
WOODS,
J.
J.
(1982).
The
absence
of
neuromuscular
transmission
failure
in
sustained
maximal
voluntary
contractions.
Journal
of
Physiology
330,
265-278.
BIGLAND-RITCHIE,
B.
R.
&
WOODS,
J.
J.
(1984).
Changes
in
muscle
contractile
properties
and
neural
control
during
human
muscular
fatigue.
Muscle
and
Nerve
7,
691-699.
12

TONIC
VIBRATION
REFLEX
IN,
MUSCLE
FATIGUE
1
BONGIOVANNI,
L.
G.,
HAGBARTH,
K.-E.
&
STJERNBERG,
L.
(1990).
Prolonged
muscle
vibration
reducing
motor
output
in
maximal
voluntary
contractions
in
man.
Journal
of
Physiology
423,
15-26.
BURKE,
D.,
GANDEVIA,
S.
C.,
MACEFIELD,
G.
&
MCKENZIE,
D.
K.
(1988).
Discharge
of
human
motor
axons
recorded
proximal
to
a
complete
block
of the
ulnar
nerve.
Journal
of
Physiology
401,
48P.
BURKE,
D.,
HAGBARTH,
K.
-E.,
LOFSTEDT,
L.
&
WVALLIN.
B.
G.
(1976a).
The
response
of
human
muscle
spindle
endings
to
vibration
of
non-contracting
muscles.
Journal
of
Physiology
261,
673-693.
BURKE,
D..
HAGBARTH,
K.
-E.,
L6FSTEDT.
L.
&
WALLIN,
B.
G.
(1976b).
The
response
of
human
muscle
spindle
endings
to
vibration
during
isometric
contraction.
Journal
of
Physiology
261,
695-7
11.
BURKE.
D.,
HAGBARTH,
K.
-E.
&
SKUSE,
M.
L.
(1978).
Recruitment
order
of
human
spindle
endings
in
isometric
voluntary
contractions.
Journal
of
Physiology
285,
101-112.
DECORTE,
L.,
EMONET-DtNAND,
F.,
HARKER,
D.
W.,
JAMI,
L.
&
LAPORTE,
Y.
(1984).
Glycogen
depletion
elicited
in
tenuissimus
intrafusal
muscle
fibres
by
stimulation
of
static
gamma-axons
in
the
cat.
Journal
of
Physiology
346,
341-352.
DE
GAIL,
P.,
LANCE,
J.
W.
&
NEILSON,
P.
D.
(1966).
Differential
effects
on
tonic
and
phasic
reflex
mechanisms
produced
by
vibration
of
muscles
in
man.
Journal
of
Neurology,
Neurosurgery
and
Psychiatry
29,
1
-11.
DELWAIDE,
P.
J.
(1973).
Human
monosynaptic
reflexes
and
presynaptic
inhibition.
In
New
Developments
in
Electromyography
and
Clinical
Neurophysiology,
vol.
3,
ed.
DESMEDT,
J.
E.,
pp.
508-522.
Karger,
Basel.
DESMEDT,
J.
E.
&
GODAUX,
E.
(1978).
Mechanism
of
the
vibration
paradox:
excitatory
and
inhibitory
effects
of
tendon
vibration
on
single
soleus
muscle
motor
units
in
man.
Journal
of
Physiology
285,
197-207.
EKLUND,
G.
&
HAGBARTH,
K.
E.
(1966).
Normal
variability
of tonic
vibration
reflexes
in
man.
Experimental
Neurology
16,
80-92.
EMONET-DtNAND,
F.
&
LAPORTE,
Y.
(1978).
Effect
of
prolonged
stimulation
at
high
frequency
of
static
and
dynamic
gamma
axons
on
spindle
primary
endings.
Brain
Research
151,
593-598.
GARLAND,
J.,
GARNER,
S.
H.
&
MCCOMAS,
A.
J.
(1988).
Reduced
voluntary
electromyographic
activity
after
fatiguing
stimulation
of
human
muscle.
Journal
of
Physiology
401,
547-556.
GRIMBY,
L.,
HANNERZ,
J.
&
HEDMAN,
B.
(1981).
The
fatigue
and
voluntary
discharge
properties
of
single
motor
units
in
man.
Journal
of
Physiology
316,
545-554.
HAGBARTH,
K.
-E.
&
EKLUND,
G.
(1966).
Motor
effects
of
vibratory
muscle
stimuli
in
man.
In
Muscular
Afferents
and
llotor
Control,
Nobel
Symposium
I,
ed.
GRANIT,
R.,
pp.
177-186.
Almqvist
and
Wiksell,
Stockholm.
HAGBARTH,
K.
-E.
&
EKLUND,
G.
(1969).
The
muscle
vibrator
-a
useful
tool
in
neurological
therapeutic
work.
Scandinavian
Journal
of
Rehabilitation
Medicine
1,
26-34.
HAGBARTH,
K.
-E.,
KUNESCH,
E.
J.,
NORDIN,
M.,
SCHMIDT,
R.
&
WALLIN,
E.
U.
(1986).
Gamma
loop
contributing
to
maximal
voluntary
contractions
in
man.
Journal
of
Physiology
380,
575-591.
HULTBORN,
H.,
MEUNIER,
S.,
MORIN,
C.
&
PIERROT-DESEILLIGNY,
E.
(1987a).
Assessing
changes
in
presynaptic
inhibition
of
Ia
fibres:
a
study
in
man
and
the
cat.
Journal
of
Physiology
389,
729-756.
HULTBORN,
H.,
MEUNIER,
S.,
PIERROT-DESEILLIGNY,
E.
&
SHINDO,
M.
(1987b).
Changes
in
presynaptic
inhibition
of
la
fibres
at
the
onset
of
voluntary
contraction
in
man.
Journal
of
Physiology
389,
757-772.
KERNELL,
D.
&
MONSTER,
A.
W.
(1982a).
Time
course
and
properties
of
late
adaptation
in
spinal
motoneurones
of
the
cat.
Experimental
Brain
Research
46,
191-196.
KERNELL,
D.
&
MONSTER,
A.
W.
(1982b).
Motoneurone
properties
and
motor
fatigue.
Experimental
Brain
Research
46,
197-204.
LANCE,
J.
W.,
DE
GAIL,
P.
&
NEILSON,
P.
D.
(1966).
Tonic
and
phasic
spinal
cord
mechanisms
in
man.
Journal
of
Neurology,
Neurosurgery
and
Psychiatry
29,
535-544.
MCCLOSKEY,
D.
J.,
EBELING,
P.
&
GOODWIN,
G.
M.
(1974).
Estimation
of
weights
and
tension
and
apparent
involvement
of
a
sensee
of
effort'.
Experimental
Neurology
42,
220-232.
MARSDEN,
C.
D.,
MEADOWS,
J.
C.
&
MERTON,
P.
A.
(1971).
Isolated
single
motor
units
in
human
13

14
L.
G.
BONGIOVANNI
AND
K.
-E.
HAGBARTH
muscle
and
their
rate
of
discharge
during
maximal
voluntary
effort.
Journal
of
Physiology
217,
12-13P.
MERTON,
P.
A.
(1954).
Voluntary
strength
and
fatigue.
Journal
of
Physiology
123,
553-564.
VALLBO,
A.
B.
(1973).
Impulse
activity
from
human
muscle
spindles
during
voluntary
contractions.
In
New
Developments
in
Electromyography
and
Clinical
Neurophysiology,
vol.
3,
ed.
DESMEDT,
J.
E.,
pp.
251-262.
Karger,
Basel.
VALLBO,
A.
B.
(1974).
Human
muscle
spindle
discharge
during
isometric
voluntary
contractions.
Amplitude
relations
between
spindle
frequency
and
torque.
Acta
physiologica
scandinavica
90,
319-336.
VALLBO,
A.
B.,
HAGBARTH,
K.
-E.,
TOREBJ6RK,
H.
E.
&
WALLIN,
B.
G.
(1979).
Somatosensory,
proprioceptive,
and
sympathetic
activity
in
human
peripheral
nerves.
Physiological
Reviews
59,
919-957.
WOODS,
J.
J.,
FURBUSH,
F.
&
BIGLAND-RITCHIE,
B.
(1987).
Evidence
for
a
fatigue-induced
reflex
inhibition
of
motoneuron
firing
rates.
Journal
of
Neurophysiology
58,
125-137.